How rocks keep time and why they might be lying
6 May 2025|Benjamin Hess
- Research
Imagine if the city of Oxford were buried beneath the towering Himalayas. How long would it take for it – or a chunk of rock that size – to rise back to the surface? You’d probably guess millions of years. But a surprising study from Holsnøy, a small island in Norway about the size of Oxford, suggests it might take just 100 years for that rock to return to near the surface. In geological terms, that’s almost instant! To understand how researchers came to this conclusion, we need to talk about minerals known as “crystal clocks”.
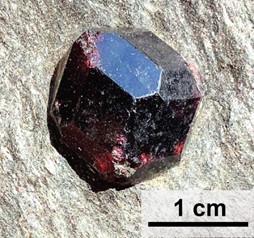
As tectonic plates collide and push rocks deep into the Earth, the intense heat and pressure can cause minerals to grow. Some of these minerals, like garnet – a small, round, red crystal – grow in layers, a bit like tree rings. Each layer, or zone, forms at a different stage of the rock’s journey underground and has its own unique chemical makeup. By slicing a garnet crystal open and analysing these zones, scientists can piece together the rock’s journey through the Earth.
Growth zones set the stage for garnets to become “crystal clocks”. At high temperatures (500–1,000 ºC or more), atoms inside the crystal start to move. They jump between the layers, slowly blending the chemical boundaries between them – a process called diffusion. The longer the crystal stays hot, the more blurred these boundaries become. By measuring how sharp or smooth the zones are, scientists can estimate how long the crystal was at high temperature. That’s why we call them crystal clocks.
Returning to Holsnøy: about 400 million years ago, this island was buried around 70 km beneath what is now Greenland and heated to about 700 ºC – similar to the depth and temperature of the bottom of the Himalayas. When researchers looked at the garnet crystals from Holsnøy, they saw very sharp boundaries between chemical zones. That suggested the rock wasn’t hot for long – maybe just a few hundred years.
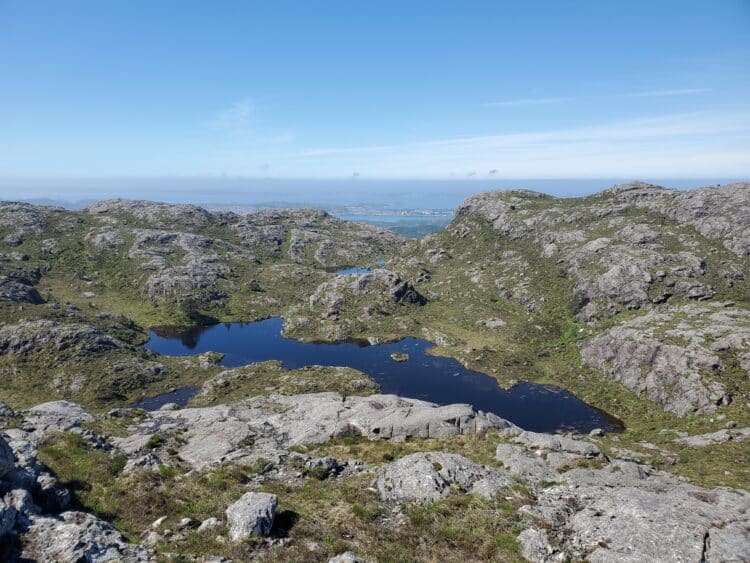
But here’s the problem: that kind of rapid cooling is hard to explain with what we know about geology. Heating and cooling a massive chunk of Earth that fast would take extreme processes – extremely rapid uplift or rapid heating and cooling from bursts of hot fluid or intense earthquakes in cold rock – but none of these ideas are consistent with our field observations and physical models.
So I asked a different question: what if the crystal clocks themselves were giving us the wrong time?
It turns out our previous models for crystal clocks made a big assumption: that the atoms moving through a crystal are all the same size. But they are not. In garnet, calcium atoms are about 10% bigger than magnesium atoms. The connections between atoms in a crystal, called bonds, are like tight springs. Calcium and magnesium atoms trade places as they move through a garnet crystal, stretching the crystal bonds in some places and squeezing them in others. This creates a lot of stress inside the crystal.
That stress changes how atoms move. The build-up of stress makes it so that the atoms are unable to fully blend or smooth the sharp boundaries between zones. They remain sharp, even if the rock stays hot for a long time!
When I included this effect in a new model and applied it to garnets from Holsnøy, the results changed dramatically. Instead of cooling in just a few hundred years, we found that the rocks likely cooled over about a million years – a much more reasonable timeline that fits with what we know about tectonic processes.
By improving our understanding of how atoms behave inside crystals, we can now use crystal clocks more accurately. That helps us better measure the timing of geological events and get clearer insights into how our planet has changed over deep time.
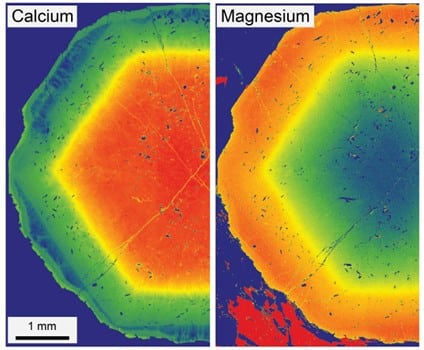
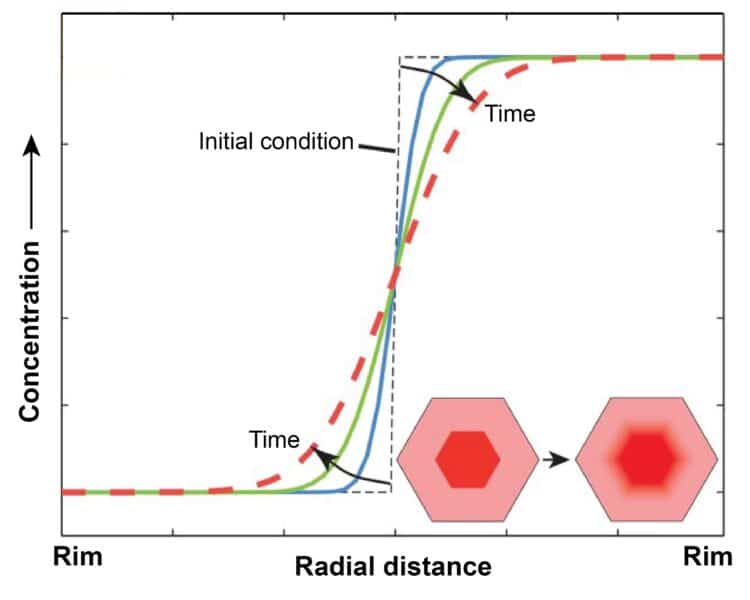
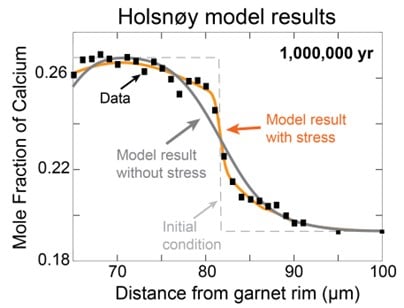
Category: Research
Author
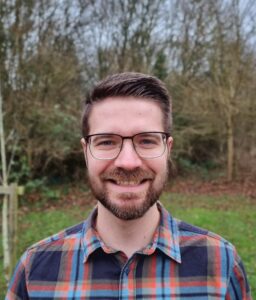
Benjamin
Hess
Benjamin Hess is an Early Career Teaching and Research Fellow in Earth Sciences at St Edmund Hall.