The Inevitable Energy Transition
10 Mar 2023|David McMeekin
- Research
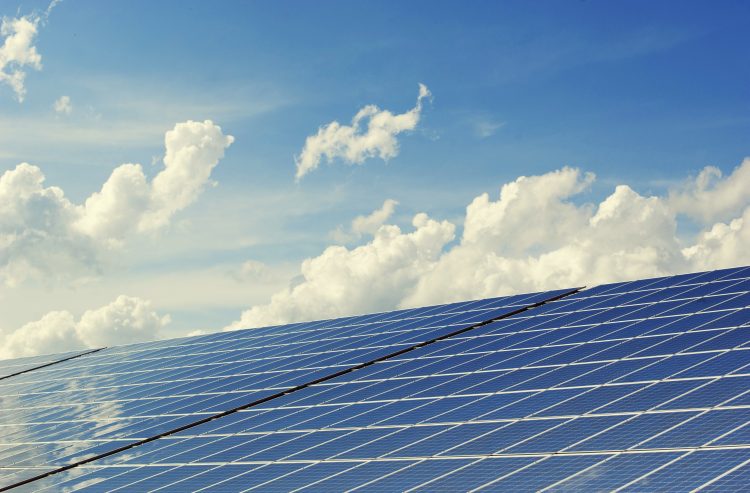
We have just marked the anniversary of the devastating and senseless Russo-Ukrainian conflict. The invasion has taken the lives of tens of thousands on both sides of the conflict and created more than 8 million Ukrainians refugees. The current conflict has caused a major shift in global and European geopolitical tensions. This led to major Russian pipelines supplying Europe with gas to be shutdown. Nord Stream 1 pipeline, which normally supplies approximately 35% of all the gas to European Union States imported from Russia, has completely closed in late August 2022. Furthermore, Germany has recently halted the certification of Nord Stream 2, which was completed in 2021. In turn, energy prices have soared due to restrictions imposed on Russian imports of natural gas and other fossil fuels. This spike in energy prices and, more importantly, the sudden realisation that Europe’s energy policies are at the mercy of a handful of countries has further emphasised the need for countries to become energetically self-sufficient. An obvious solution to this energy catastrophe partially resides in a transition towards sustainable and renewable energy sources. Solar energy is the most abundant energy resource on earth and available in every country.
Transitioning towards renewable energies has previously been difficult due to the need for direct or indirect government subsidies to support initial adoption of emerging technologies. However, this is no longer the case, where unsubsidised utility-scale bids for wind and solar projects have come in at lower price ranges than gas or coal projects, which have long been regarded as the lowest Levelised Cost of Energy (LCOE). According to the Lazard’s report, utility-scale renewables are now below coal generation prices, where unsubsidised wind prices ranged from 28 to 54 USD/MWh, while utility-scale solar ranged from 32 to 42 USD/MWh, compared to coal priced at 66 to 152 USD/MWh, and gas combined cycle at 44 to 68 USD/MWh. Enabling this cost-competitiveness has triggered an inevitable energy transition from fossil fuel-based technologies to renewables. This transition is no longer an environmentalist’s pipe dream, but rather a current reality, which now has been accelerated by the soaring energy cost caused by the current geopolitical conflict.
A viable, abundant and now relatively cheap source of energy is solar power. To date, crystalline silicon-based (c-Si) photovoltaics have dominated the solar market. Although c-Si-based solar cells have been around for many decades, they have only been truly cost-effective in the most recent decade. In the late 1990s, universities, research institutes and industrial partners have developed crystalline silicon technology to reach a 25% power conversion efficiency milestone. From that point forward, the world record efficiency has only risen to 26.7%, as we are approaching a theoretical limit of 33.16% for a single-junction solar cell, known as the “Shockley–Queisser limit”. Hence, it is becoming increasingly difficult to improve the efficiency of the conventional crystalline silicon solar cell. Recent cost reductions in solar energy have mainly been achieved by advancements in manufacturing techniques and reaching economies of scale. As silicon-based solar cells efficiencies converge towards their maximum theoretical limit, price reductions will soon become increasingly difficult to achieve. However, multi-junction architectures can overcome the single-junction efficiency limit, by absorbing distinct portions of the solar spectrum using materials with different band gaps, leading to an increase in solar cell efficiency. These architectures allow for a reduction in photon thermalisation losses; a loss mechanism that occurs when a high energy photon partially converts its energy to heat instead of transferring it to an electron. Multi-junction solar cells are formed by mechanically stacking, on top of one another, two or more solar cells with different band gaps, allowing each junction to absorb different portions of the solar spectrum. A relatively small increase in performance, obtained by transitioning towards multi-junction architectures, could lead to significantly lower solar energy prices, since the other major costs such as land rights, installation, and inverters should remain constant.
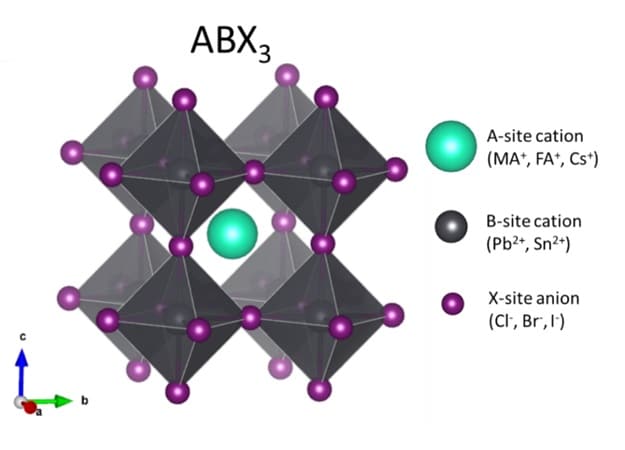
A strong candidate for multi-junctions are metal-halide perovskite solar cells, which form in a generic ABX3 crystal structure, where the A-site is typically an organic or inorganic cation, the B-site is a divalent metal cation, and the X-site a halide anion. Although perovskites materials have been discovered since the 19th century, perovskite structures have only emerged for solar application in the last decade or so. Oxide-based perovskite crystal structures occur abundantly in nature, with approximately 38% of the earth’s volume forming in a perovskite crystal structure. However, here in the Clarendon Laboratories, we focus our efforts on researching and developing halide-based perovskites, which form crystal structures that are particularly good at absorbing light, while maintaining long charge-carrier lifetimes with the semiconductors. Furthermore, we can fabricate metal-halide perovskite solar cells with various band gaps by tuning their chemical composition. The band gap of a semiconductor represents the energy required to promote an electron from the valence band to the conduction band, thus creating a “free-electron” that can move freely within the crystal lattice. This process occurs after a photon with enough energy gets absorbed by the perovskite material. Using specific materials or dopants, these free-electrons can then be extracted at their respective electrodes to create photogenerated current and voltage.
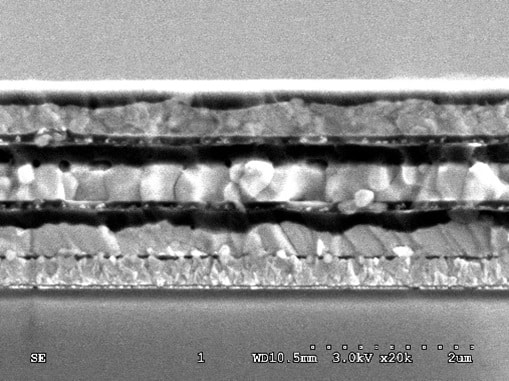
The ability to tune their band gap makes metal-halide perovskite solar cells a perfect candidate for multi-junction solar cells. Perovskite-silicon tandems and/or all-perovskite multi-junction solar cells, may certainly be the next technological advancement in the photovoltaic roadmap, however, their long-term stability has yet to match conventional silicon-based technology. Hence, in the photovoltaic and optoelectronic group, located in Oxford’s Condensed Matter Physics (CMP) department, we are constantly trying to find new perovskite materials, architectures, or deposition techniques that improve the overall stability of these solar cells to make them commercially viable.
As the cost of photovoltaic energy has plummeted, countries now have the option to lessen their dependence on fossil fuels, along with the countries that control these resources. Energy self-sufficiency has become a major policy concern since the start of the Russo-Ukrainian conflict. The energy policy change from non-renewable fossil-fuel based sources towards low carbon emission-based renewables is inevitable considering the economic advantages renewables now have to offer. Hence, in my opinion, along with the emergence of cheaper energy storage, it is not a question of “if”, but rather a question of “when” the world will change to a combination of renewable energy sources. However, citizens, politicians and scientists can significantly accelerate this change to mitigate the impact of climate change.
Category: Research
Author
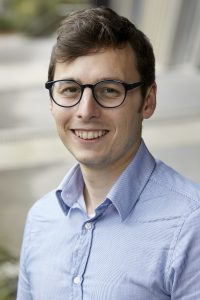
David
McMeekin
David is a Junior Research Fellow in Physics and a Marie Skłodowska-Curie Fellow at the University of Oxford.