What starts Alzheimer’s disease?
19 May 2021|Paul Matthews
- Research
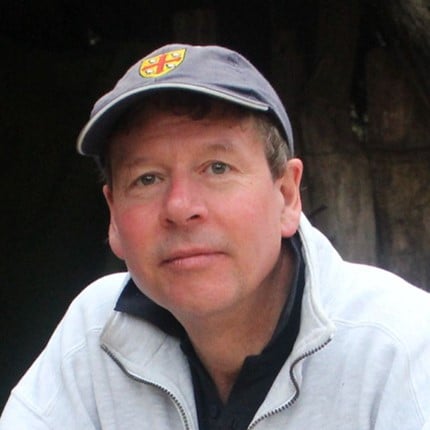
My laboratory in the UK Dementia Research Institute at Imperial College London is working to understand what the first events in the disease are – at the stage when expression of disease can only be detected inside the cells of the brain. Discovery of genes associated with susceptibility to AD over the last decade provides the foundation for an exciting new strategy to develop this understanding. I call it a “gene first” approach. In this, I reason that cellular pathways including proteins made from genes associated with AD susceptibility give us a clue to those involved in the earliest stages of the disease, defining those pathways that are able to trigger initiation of the cascade of pathology that, when it progresses unchecked, leads to AD. By using genes to guide us, we can study these pathways even using brain tissue obtained from patients after death.
Even so, brains are complicated! They are built from nerve cells of different types and even more abundant glial cells. One of the most unexpected discoveries of the last few years was that the brain cell that expresses the greatest number of AD genes is the smallest of the glial cells, the microglia. This argues that Alzheimer’s disease begins not in the nerve cells, but here, in these tiny glial cells. Early pathology may be found only secondarily in nerve cells. If the treatment could be found to slow or stop the early pathology in microglia, perhaps AD could be prevented!
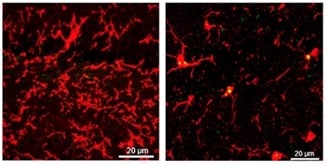
I am studying microglia and their interactions in brain tissue using a range of “’omics” technologies- genomics, which tells us which AD risk genes are carried and, for each cell individually, transcriptomics to define those genes which are copied to make proteins, epigenomics, which informs how the signals regulating which genes are copied (or how much they are copied) for protein synthesis are controlled, and protein expression. We can do these studies on brain tissue from people with AD and from those without any brain disease. Comparing them lets us know what is happening with disease, in which cell and where in the brain. However, we also need to know why the programme of disease starts.
What is most striking about AD is that, while it is different from healthy aging, it is a disease of aging. I therefore hypothesise that the interaction of AD genetic risk with cell senescence (a fancy term for cell aging) is somehow responsible for triggering the disease programme. A candidate mechanism is oxidative damage – cellular “rusting”. To understand why I are interested specifically in this process, let me highlight a disease analogy: atherosclerosis is driven by macrophages (cells very similar to microglia that are found mainly outside of the brain), which damage the wall of a large blood vessel (leading to subsequent local blood clot formation) after they take up circulating fats in the blood that have been oxidised (“rusted”).
The genetics of AD risk and its disease association suggests that this correspondence may go further than simple analogy. A number of the genes determining AD risk have roles in the transport of fats in and out of microglia. Moreover, people with diabetes, which disturbs a healthy balance of fats in the body, have a higher risk of AD than the healthy population. Is it possible that there is a potent, toxic interaction between microglial genetic susceptibility, aging and a long-term unhealthy balance of blood fats in the body that triggers initial steps in AD?
A number of scientists around the world and I are excited by this idea because it suggests that treatments previously designed for people with diabetes (preventing an unhealthy balance of blood fats) could be used to prevent AD! In fact, the diabetes drugs liraglutide and exenatide already are being tested for their potential to slow AD. There also are some remarkable ideas continuing to emerge about ways of slowing cell senescence. I hope that soon some of these may enter clinical trials for AD soon.
Of course, other ways of preventing or treating AD also are being pursued. Most of the high profile effort thus far has been put into use of large proteins (antibodies) that capture and wash the toxic protein amyloid-beta from the brain. While one of the most recent, adacanumab, shows promise (and may be approved for patients to use this year), results from these approaches have been disappointing. My guess is that amyloid becomes involved somewhat later in the cascade of events that I have described, so, if they are still pushing the disease forward, washing amyloid out may have a small effect.
I do not have the answers, but I wake everyday with optimism that – despite having no meaningful treatments for patients today – the scientific community is poised to make progress rapidly. Clinical science of the brain has reached an inflection point on its trajectory upwards!
Category: Research
Author
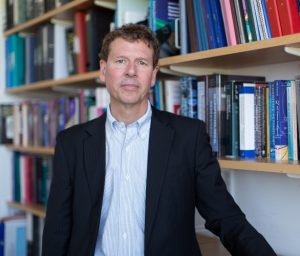
Paul
Matthews
I am clinical academic. My research focuses on Alzheimer’s disease (AD). I came to study this as I learned first-hand how terrible this disease is, both as a neurologist and as a witness to a family member succumbing. AD is insidious in its onset, beginning slowly (and initially asymptomatically), decades before people complain of memory difficulties.