Microbes and the Biological World
2 Jun 2021|Wei Huang
- Research
Microbes have pervasive, remarkably profound, and generally positive effects on human health and the entire biological world. Microbes are the life support system of the biosphere. Millions of years ago, cyanobacteria produced oxygen, heralding the explosive evolution of life on earth. Even now they still make a significant contribution to carbon recycling in the global biogeochemical system. We are only half human if we count cells of our body one by one. There are 30 trillions of bacteria in a human, equivalent to the total number of cells in a reference human (70 kilograms, 1.7 m tall). Most human-associated bacteria are living in the gut, processing food, modulating our health, and adjusting our mood by forming gut-brain axis via sending signals to communicate with the brain. Bacteria usually colonise in human tumours, and the composition of bacteria varies from tumour to tumour. I wonder if both our mood and health are controlled by these tiny bugs. Microbes can make various medicines, such as anti-cancer drugs and antibiotics. They help the production of various foods and drinks, such as bread, yoghurt, beer, and wine. They also maintain soil fertility by making ammonia from nitrogen-fixing bacteria, and they support the ecosystem by nursing the forest from mycorrhizae. In addition, microbes also clean our wastewater, degrade contaminants, and recycle biological wastes.
Generally microbes can be regarded as fast-growing and self-replicating cell factories. With the advances on synthetic biology and molecular biology, microbes will play an important role in boosting the global economy and curbing global CO2 emissions, which will designate the 4th Industrial Revolution. Synthetic biology is the application of engineering principles to design cells for novel tasks. We can engineer simple microbes to achieve sustainable development (e.g. green chemical engineering using CO2), improve agriculture activity (e.g. increase crop yields), biosynthesise novel medicines and develop new medical therapies. In 2016, the US National Science and Technology Council has published ‘Advanced manufacturing’, predicting that microbes designed by synthetic biology can lead to the eighth manufacturing – advanced biomanufacturing.
A biological cell is a computational system, comprised of software (genomic DNA) and hardware (machinery of life, such as RNA polymerase, and ribosomes to read and process the genetic code). The central dogma of a cell is DNA>RNA>protein. This is like the information processing of a computer: codes in hard disk>RAM>display. DNA and the hard disk both store the original code. RNA transcribes working code from DNA, just like how RAM reads and stores working data from the hard disk. Synthetic biologists also borrow the principles of logic gates and electronic circuits to design genetic gates and gene-circuits of a cell. Perhaps the only difference between a cell and a computer is that the software of a cell determines its hardware. Once the first machinery of life (hardware) is made, the genetic code (software) can instruct the hardware to copy itself. Hence, an engineer only needs to design genetic codes without constructing the hardware of a cell.
However, when a synthetic biologist genetically attempts to modify a microbe, we encounter a conflict of interest between life and human will. The aim of a cell is to survive and replicate itself. The goal of synthetic biology is to engineer a cell to perform our wishes. The novel tasks assigned to cells usually incur metabolic burdens, which are not helpful to cell survival and reproduction. Cells such as bacteria have been evolved for billions of years. Their genetic codes are made for survival, which will inevitably interfere with attempts by researchers to engineer the cell. This inherent conflict can lead to unpredictable performance, or crash of synthetic gene-circuit designs.
Since synthetic biology is to engineer cells for novel tasks, it inevitably involves in genetic modification. A challenge synthetic biology faces is the potential negative consequences of genetically modified organisms (GMOs) and public concerns.
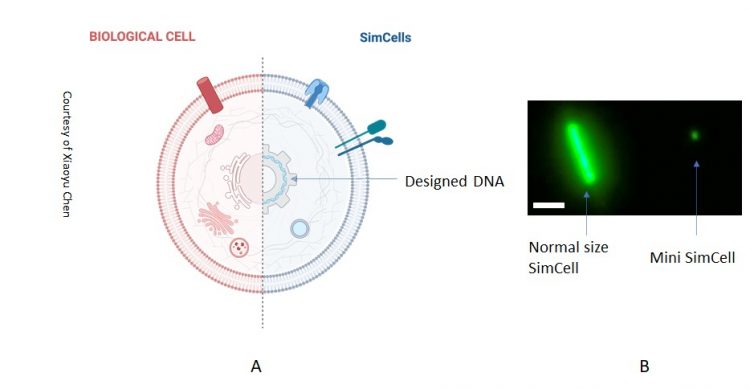
SimCells remove the chromosomes of bacteria and replace them with designed genomes. It is like a computer that has been reformatted and installed with a new operating system. A SimCell is an artificial cellular machine, inheriting the machinery of a cell and replacing the native genome with a designed gene-circuit or a minimal genome. Its intact cellular machinery faithfully reads instructions encoded in engineered gene-circuits without the interference from native gene networks. A SimCell is a non-living entity, unable to reproduce and highly controllable. It can be regarded as smart bioparticles. Hence, SimCell application has no issues concerning GMOs.
To demonstrate the utility of SimCells, we apply them to cancer treatment. There are two types, mini and normal SimCells (Figure). The size of a mini SimCell is 100-400 nm, small enough to penetrate the loose tumour tissue. SimCells are designed to specifically bind onto cancer cells by recognising the biomark proteins on their surface. Then, SimCells will be induced by aspirin to make anti-cancer drugs, locally creating a high concentration of potent catechol to kill cancer cells. After locating and landing on cancer cells, SimCells can also send a signal to call T cells in the human immune system to attack the cancer cells.
Since SimCells are chromosome free, we can install DNA at different levels, from a simple gene circuit to an artificial genome. By creating this new software to SimCells, we can interrogate some fundamental questions about the nature of life.
Category: Research
Author
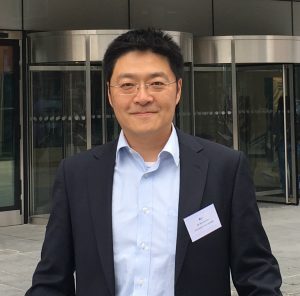
Wei
Huang
Professor Wei Huang is a Professor of Biological Engineering at the University of Oxford, working on synthetic biology and Raman single cell biotechnology. He is an EPSRC fellow in Synthetic Biology.