An Open and Shut Case: Membrane Transport in Health and Disease
4 Feb 2019|Robert Wilkins
- Research
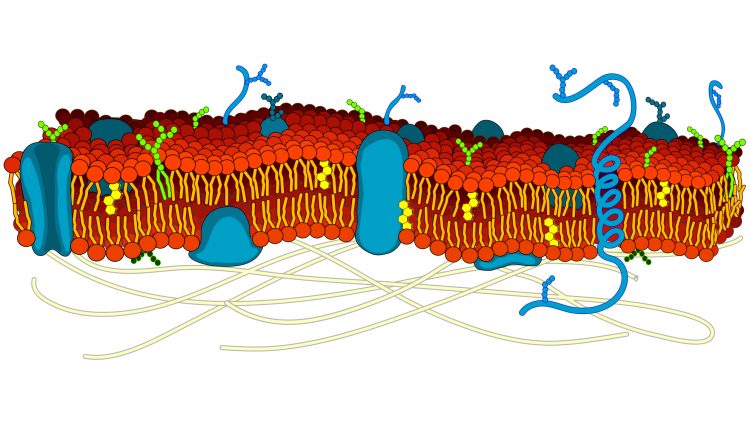
There are three types of protein in cells that can be the target for drugs: receptors, enzymes and transporters. While inhibitors of receptor proteins (‘Beta blockers’) and inhibitors of phosphodiesterase enzymes (‘Viagra’) are well known, the function of membrane transport proteins is less familiar but equally important.
My interest in membrane transport proteins was sparked when I first learnt about them as an undergraduate over a quarter of a century ago. From almost the first lecture, transport proteins featured regularly and it rapidly became clear that they had a fundamental role in physiological function. One of my tutors was an expert in the field of membrane transport, so our discussions in tutorials regularly led us to consider what these proteins do and how they do it. Enthused by those discussions, my work at Finals focused on transporters and I had my first taste of laboratory research, characterising the systems regulating the levels of acid in cartilage cells. A D.Phil building on that work followed, leading to a research career that has investigated the possible involvement of membrane transport proteins in two disease states: arthritis and cancer. We’ll come back to that work at the end of this blog, but first let me explain the basics of membrane transport…
The 35 trillion cells in the human body vary greatly in appearance, location and function, but they all possess a membrane, which separates the fluids inside and out. The membrane is a flexible ‘sea’ of lipid molecules, in which float a variety of protein molecules.
Some of the proteins act as markers (‘recognition signals’) to identify the cell type; others are docking stations (‘receptors’) for chemicals such as hormones and neurotransmitters that instruct the cell what to do; some are enzymes, catalysing reactions that create chemical messages to convey those instructions; yet others act as transporters (‘channels’ and ‘carriers’) to allow for movement of solutes (ions and organic molecules such as glucose) between the cell and its surroundings.
This last category is needed because most of the chemicals relevant to cell function don’t readily cross the lipid membrane. While gases such as oxygen and carbon dioxide can dissolve in the lipid membrane and so move into or out of the cell, metabolites and ions need assisted passage. Membrane transport proteins therefore play essential roles in cellular function: their actions underpin nerve impulses, muscle contraction, the heartbeat, urine formation and absorption of food in the gut. Perhaps unsurprisingly, inadequate or excess activity of these proteins leads to disease, while a number of common drugs exert their actions by modifying transporter function.
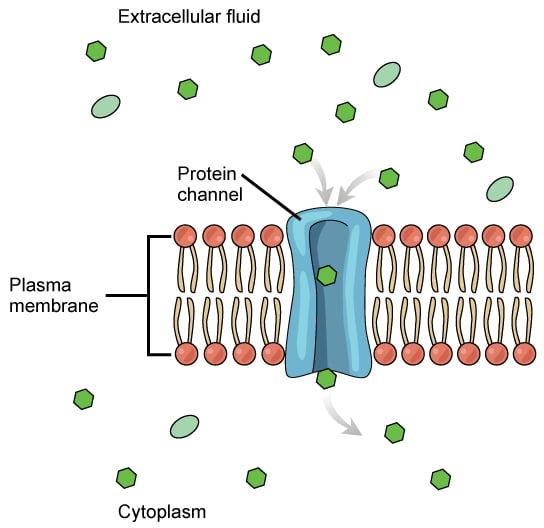
Channels are like doors…
Transporters are effectively ‘doors’ in the ‘wall’ that is the cell membrane, through which solutes can move. The simplest transporters – leak channels – are like a door frame that lacks a door connecting the two sides of the membrane. Solutes move from the side where they are plentiful to the side where they are in short supply, but no control can be exerted over how much traffic occurs across the membrane. Malaria parasites in infected red blood cells may subvert these channels to establish a pathway to gain nutrients from outside the cell and expel the waste products of their metabolism.
In a gated channel, there is a door within the frame that can be open or closed, providing regulated traffic. The opening of ion channels sensitive to voltage is fundamental to nervous signaling and the electrical activity that creates the heartbeat: defects in the function of voltage-gated channels in the heart can lead to cardiac arrhythmias. Channels that are gated by neurotransmitter binding allow nerve-to-nerve signaling and convert nervous impulses into muscle contractions, while the opening of channels when the membrane of tendon cells is stretched initiates the knee jerk reflex.
Other channels control the secretion of saliva and of mucus in the lung. In the genetic disease cystic fibrosis, a channel protein that allows the passage of chloride ions is faulty. The movement of chloride is needed to draw water into the airway, without which the protective lining is too sticky and the airway becomes prone to infection.
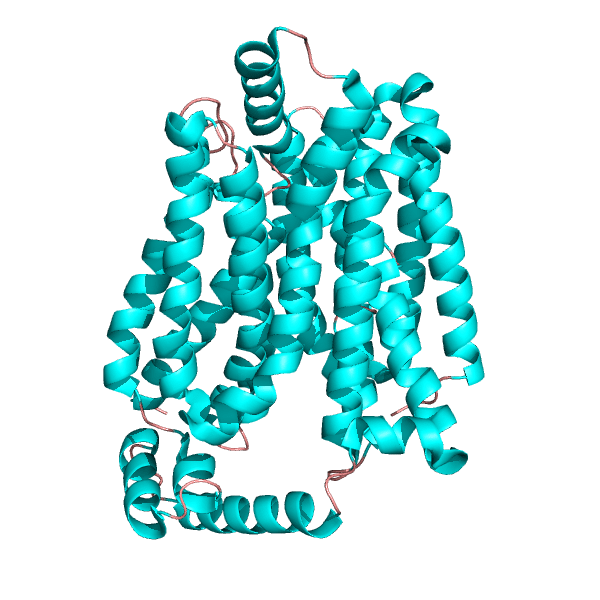
…and carriers are like revolving doors
Carrier proteins function like revolving doors and, just as we can be conveyed through a revolving door by someone pushing from behind or coming in the opposite direction, so some of these transporters can use the movement of one solute to drive the flow of another against its natural tendency, so leading to accumulation inside, or extrusion from, the cell. Many of these transporters fulfil ‘housekeeping‘ functions for cells, exporting wastes and maintaining cell size. Red blood cells in sickle cell disease patients show abnormal housekeeping, which increases the likelihood that the cells will clump in blood capillaries. Transporters of this type are essential for scavenging glucose and amino acids in the gastrointestinal tract: defects lead to nutrient malabsorption. In the kidney, their action allows the body to vary the composition of the urine in response to altered salt and water intake. ‘Water tablets’ (aka diuretics such as furosemide and chlorthiazide) exert their effects by inhibiting carriers that move sodium ions across the membranes of renal cells. Some antidepressants (for example, ‘Prozac’) work by inhibiting transporters for neurotransmitters, increasing levels of these signalling molecules in the brain to alter mood; cocaine also exerts some of its actions through effects on transmitter transporters.
The most complicated transporters are like electrically operated revolving doors, where the door rotates without us needing to push on it. These ‘active pumps’ use the cell’s energy store to power ion movements and create asymmetric distributions of ions on either side of the cell membrane. The asymmetry creates gradients and, when ions move down those gradients on carriers, the ‘push’ on the door provides the drive that enables other solutes to be accumulated or expelled as already described. This powered transport also underlies the secretion of acid in the stomach, which is important for the digestion of ingested proteins: the drug omeprazole, used to treat gastric reflux and ulcers, inhibits this pump.
Arthritis and Cancer
My research has focused on two proteins that perform housekeeping roles in cells and their possible involvement in disease. The first protein is the Na+-H+ exchanger (NHE), which is one of the transporters that regulates the levels of acid (the pH) in cells. In cartilage, chondrocytes are responsible for the upkeep of the extracellular matrix that surrounds them and acts as a cushion between the bones in joints. My work showed that the level of acid inside chondrocytes influences the production of new cartilage. I also showed that chondrocytes rely almost exclusively on NHE to regulate their pH. Therefore, anything that changes the activity of this protein can lead to altered levels of cartilage synthesis through changes in pH: chemicals that are found at higher levels in arthritic joints have such effects. This marks out the carrier protein as a potential pharmaceutical target to promote synthesis of new cartilage.
The second protein is the K+-Cl- cotransporter (KCC), which is one of the transporters by which cells regulate their volume. With colleagues in Oxford and Taiwan, I have investigated how cancer cells use KCC to defend themselves from swelling and bursting when water enters them. Increased numbers of KCC proteins in cancer cells promotes the proliferation and invasion of these cells in tissues such as the cervix. Understanding how KCC does that could open up new approaches for limiting cancer development and spread in those tissues.
These are just two examples of the ways in which this array of proteins, with diverse distribution and function in the body, are being recognised as targets for drugs, prospects for gene therapy, and even as possible routes of drug delivery in the intestine.
Read more on our blog
Category: Research
Author
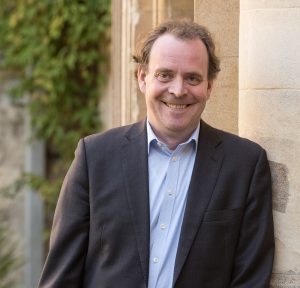
Robert
Wilkins
Professor Robert Wilkins holds the American Fellowship in Physiology at St Edmund Hall. He oversees the admission, teaching and pastoral support of Biomedical Sciences and Medicine students and provides tutorials covering cellular and systems physiology.